Effects of Invasive Species on Water Quality in Freshwater Ecosystems by Laura Heller '20
Through globalization, Earth’s biomes are becoming increasingly homogenized. The introduction of invasive species creates environmental disturbances and leads to ecological degradation, which in the case of aquatic ecosystems leads to marked decreases in water quality.
The common vilification of exotic species is not necessarily justified in the sense that these “biological invasions” are common in nature. However, humankind’s acceleration of these biological introductions has the potential to accomplish a level of biome homogenization and ecosystem collapse that has never been seen. This paper will focus on the relationship between aquatic biological invasions and water quality of the invaded ecosystem, explaining why this relationship exists and what efforts should be made to mitigate these effects.
Water quality is affected by both the diversity and abundance of species and characteristics of specific species that live within or near aquatic environments, therefore management actions taken to control these plants and animals are very important. An example of this importance is in the case of the non-native stocked fish, rainbow trout, hybridizing, with the native, closely related, Alvord Redband Trout (McCormick). These two species continued to cross breed, resulting in the extinction of the Alvord Redband Trout in the wild. Other fish, such as the Red Shiner, which is highly competitive and aggressive, also led to the decline of native fish through hybridization, competition, and the introduction of pathogens (McCormick). The reason these alterations in biological diversity are important is because they can lead to what is known as a trophic cascade, where changes in the food chain affect trophic relationships and ultimately change the relative populations of predator and prey resulting in shifts in ecosystem structure and nutrient cycling (Baxter et al. 2004; Eilers et al.2007).
One such example of an invasive species affecting nutrient cycling is water hyacinth in Lake Caohai in China. Water hyacinth is an aquatic plant that tends to replace existing aquatic plants and form interlocking mats of water hyacinth, when they over grow an ecosystem they also decompose at a faster rate than the surrounding plants. This decomposition greatly affects nutrient cycles (Villamagna and Murphy 2010).
The nutrient cycle describes the use, movement, and recycling of nutrients in the environment, and is one of the most important processes that occur in an ecosystem. Elements such as carbon, oxygen, hydrogen, phosphorus, and nitrogen are essential to life and are “recycled” on both a global and local level, allowing for organisms to live. Nutrient cycles involve living and nonliving components and include biological, geological, and chemical processes.
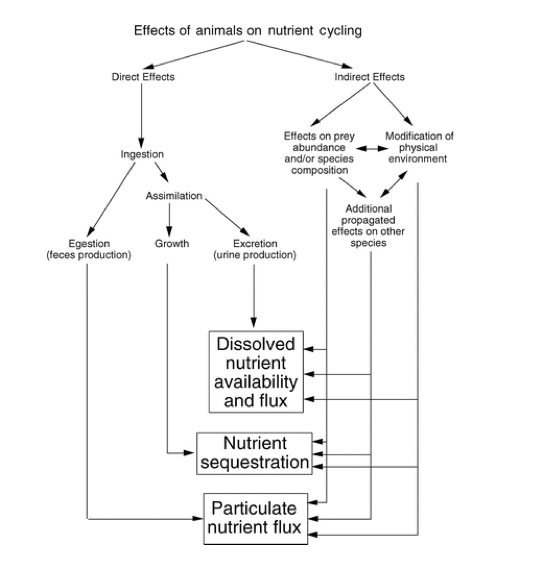
Nutrient cycling through an animal’s body can be divided into two functionally distinct processes: nutrient recycling and nutrient translocation (Vanni 2002). Nutrient recycling occurs when an animal releases nutrients within the same habitat in which food was ingested, meaning the nutrients remain in the same ecosystem. A specific example of this is when zooplankton consume phytoplankton in a lake and then excrete nutrients back into the water, they recycle nutrients that were already in that habitat. Whereas, nutrient translocation is the process where an animal physically moves nutrients between habitats or ecosystems, often accompanied by transformation of nutrients from one chemical form to another (Kitchell et al. 1979, Shapiro & Carlson 1982, Vanni 1996). A prime example of this occurrence is when an animal feeds on benthic prey and excretes the nutrients into the water. This translocates the nutrients from a benthic to a pelagic habitat, and converts nutrients from particulate to dissolved forms (Vanni 2002). In this case, animals move nutrients between habitats within a single ecosystem, but nutrient translocation can also occur between different ecosystems.
What differentiates nutrient translocation from recycling is that through translocation nutrients are moved across boundaries or against physical processes that impede nutrient movement (Vanni 2002). This interruption can have a multitude of effects on the sediment-water interface, the thermocline that separates surface and deep-water layers, and the downstream flow of water. In many aquatic systems, primary producers cannot use nutrients in deep waters because of the lack of light (Vanni 2002). Therefore, any process that brings nutrients to the euphotic zone (surface waters where light intensity is sufficient for photosynthesis) is potentially important (Vanni 2002). Translocated nutrients can stimulate “new primary production” and increase the total mass of nutrients in the recipient habitat or ecosystem (Vanni 2002). Nutrient recycling cannot directly increase the mass of nutrients in a habitat or ecosystem, but instead it sustains the nutrients in the ecosystem. The implications of whether animals recycle or translocate nutrients is important, and when invasive species are introduced the nutrient cycle is disrupted.
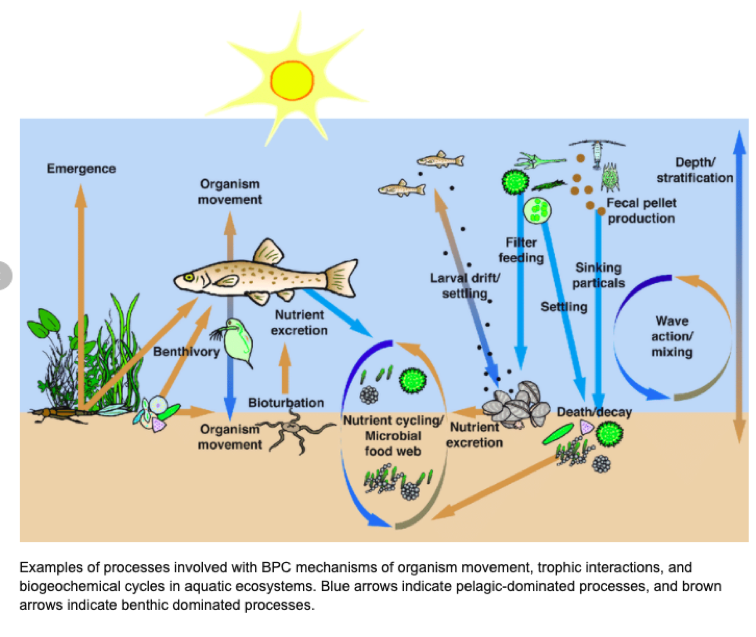
In addition to altering nutrient cycling, the transportation of invasive species can also spread diseases. The introduction of brown trout in the early 1900s into the United States from Europe led to the spread of the highly infectious Whirling disease. This disease is spread via fish, humans, dogs, birds, boat trailers, and basically any equipment that has been in infected water (McCormick). Once this parasite enters the body of the fish, it continues to survive as spores for up to 30 years, even in dried-up streambeds affecting not only fish, but there were also reports of reduced amphibian populations in montane lakes where the infected trout had been stocked (McCormick). This is one specific example of invasive species bringing parasites with them, but this is a relatively common occurrence, and as in the case of Whirling Disease, certain diseases can survive outside of the body and spread on to other species and even ecosystems. This is particularly important in riparian ecosystems, as water flows downstream leading to a larger body of water such as a lake or ocean, the introduction of parasites and diseases to other bodies of water and ecosystems could potentially disrupt entire food chains, and nutrient cycles. Another example of this is Aeromonas hydrophila, a bacteria commonly found in freshwater ponds but is fatal to fish and amphibians. Although this is considered a pathogen of fish and amphibians, there have been cases in which Aeromonas hydrophila has survived within freshwater and was then ingested by humans, causing diarrheal disease (Simmons and Gibson, 2012).
In addition to affecting health, invasive species also affect natural physical processes by altering the quantity and timing of runoff, erosion, sedimentation, and may affect water availability. Species, such as salt cedar, consume more water than their native counterparts, which subsequently lower the water table and potentially dry up streams (Wiesenborn,1996). Alternatively, invasive species may lead to increased flooding or soil moisture because of reduced transpiration, leading to increased runoff flowing into streams (McCormick). Introduced species may not just alter the amount of runoff, but also the timing of runoff arrival. If soil moisture remains higher, then there is a lower capacity for soil absorption, leading to a larger amount of runoff, and an increased likelihood of flooding. On the other hand, increased plant coverage provides “roughness” which can reduce runoff.
Invasive or non-native species degrade water quality by decreasing water flows and reducing the transportation of nutrients or by increasing runoff and erosion leading to hyper-eutrophication. The replacement of bunchgrass by knapweed increased erosion and led to an increase in stream temperatures which killed off native fish and the surrounding habitat (Lacey et al. 1989). Whisenant, also proved that Cheatgrass shades out nitrogen fixing soil crust and decreases the nitrogen input to the ecosystem (Whisenant,1990).
The primary role of nitrogen in freshwater ecosystems is as one of the key nutrients, including carbon, phosphorus, and silica, required for primary production by plants and algae in the process of photoautotrophy (Durand 2011). As invasive species affect the nitrogen cycle, as seen in the case of Cheatgrass, necessary algae and higher plants are greatly affected, altering the food web structure as microbial, algal, and plant uptake of nitrogen is changed (Durand 2011). Any change in nitrogen supply to a body of water will cause changes in that ecosystem, in the case of increased nitrogen the process of eutrophication occurs, leading to an accelerated growth of plants and algae, a disturbance to the balance of organisms, and to the quality of water (Durand 2011).
The eutrophication process can result in losses in biodiversity, a dominance of nitrogen fixing cyanobacteria, or a shift in food web structure. The added decomposition load of invasive plants also alters the loading cycle of nitrogen and phosphorus; in addition, the microbial decomposition of plant biomass decreases dissolved oxygen. Invasive species have the potential to completely alter water quality even through filtering particles from the water column. Zebra mussels filter particles from the water, however the nutrients are then concentrated in their feces which enriches the sediment and reduced turbidity which many native species rely on (Bruner et al. 1994).
Beyond shifts in water quality and biological diversity, these changes also have economic impacts. In Oregon, Tui chub, a small forage fish, was introduced which led to a drastic decrease in zooplankton and caused the growth of several forms of algae, including blue green Anabaena sp. Algae. This released the toxin anatoxin-a which led to a loss in fishing opportunity, affecting water quality, overall recreation use, and public health concerns (Eilers et al. 2007). It cost the state over $5 million to get rid of the Tui chub, by poisoning the entire lake (Opb).
Aquatic invasive species have the potential to disrupt nutrient loadings as seen with water hyacinth and bunchgrass, clean sediments like zebra mussels, nutrient cycling as observed with the redband trout, and toxin pollutants like the release of anatoxin as a result of the Tui Chub introduction. Invasive species also alter pollutants through bioaccumulation and the introduction of other contaminants into waterways. As new invasive species are introduced, many management efforts are relying on pesticides to control invasive species spread, however this mitigation strategy exacerbates ecological and human health effects. To understand the entire portfolio of ecosystem effects that non-native species have, there needs to be a systematic identification of fundamental processes in undisturbed ecosystems to more successfully evaluate both the direct and indirect effects and responses of the systems affected by biological invasions. There also needs to be an effort to determine invasive species and their effects across trophic levels world-wide, and develop a risk analysis to determine the priority of land types and wilderness.
First and foremost, it is important to prevent the introduction of invasive species. According to the EPA, more than one-third of all states in the United States have waters that are listed for invasive species under the Clean Water Act (McCormick). The issue of invasive species reaches beyond a shift in predator-prey and food chain relations and goes on to affect water quality.
Citations
Baustian, Melissa & Hansen, Gretchen & De Kluijver, Anna & Robinson, Kelly & Henry, Emily & Knoll, Lesley & Rose, Kevin & Carey, Cayelan. (2014). Linking the bottom to the top in aquatic ecosystems: mechanisms and stressors of benthic-pelagic coupling. 10.4319/ecodas.2014.978-0-9845591-4-5.38.
Baxter, C.V.; Fausch, K.D.; Murakami, M.; Chapman, P.L. 2004. Non-native stream fish invasion restructures stream and forest food webs by interrupting reciprocal prey subsidies. Ecology. 85: 2656–2663
Brown, L.R.; Moyle, P.B. 1997. Invading species in the Eel River, California: successes, failures, and relationships with resident species. Environmental Biology of Fishes. 49: 271–291.
Bruner, K.A.; Fisher, S.W.; Landrum, P.F. 1994. The role of the zebra mussel, Dreissena polymorpha. In: Contaminant cycling:II. zebra mussel contaminant accumulation from algae and suspended particles, and transfer to the benthic invertebrate,
Gammarus fasciatus. Journal of Great Lakes Research. 20:735–750.
Durand, Patrick & Breuer, Lutz & Johnes, Penny & Billen, Gilles & Butturini, Andrea & Pinay, Gilles & Grinsven, Hans & Garnier, Josette & Rivett, Michael & Reay, Dave & Curtis, Chris & Siemens, Jan & Maberly, Stephen & Kaste, Øyvind & Humborg, Christoph & Loeb, Roos & De Klein, Jeroen & Hejzlar, Josef & Skoulikidis, Nikos & Wright, Richard. (2011). Nitrogen processes in aquatic ecosystems.
Eilers, J.M.; Loomis, D.; St. Amand, A., et al. 2007. Biological effects of repeated fish introductions in a formerly fishless lake: Diamond Lake, Oregon, USA. Fundamental and Applied Limnology (Archiv fur Hydrobiologie). 169(4): 265–277
McCormick, Frank H.; Contreras, Glen C.; Johnson, Sherri L. 2010. Effects of nonindigenous invasive species on water quality and quantity. In: Dix, Mary Ellen; Britton, Kerry, editors. A dynamic invasive species research vision: Opportunities and priorities 2009-29. Gen. Tech. Rep. WO-79/83. Washington, DC: U.S. Department of Agriculture, Forest Service, Research and Development: 111-120.
Michael J. Vanni. Nutrient Cycling by Animals in Freshwater Ecosystems Annual Review of Ecology and Systematics 2002 33:1, 341-370
Simmons, J., & Gibson, S. (2012). Nonhuman Primates in Biomedical Research. American College of Laboratory Animal Medicine, 2, 105-172. doi:10.1016/c2009-0-01851-0
Whisenant, S. 1990. Changing fire frequencies on Idaho’s Snake River Plains: ecological and management implications. In: McArthur, E.D.; Romney, E.M.; Smith, S.D.; Tueller, P.T., eds. Proceedings, Symposium on Cheatgrass Invasion, Shrub Die-off and Other Aspects of Shrub Biology and Management. Las Vegas, NV. 1989. Gen. Tech. Rep., INT-2767. Ogden, UT U.S. Department of Agriculture, Forest Service, Intermountain Research Station: 4–10
Wiesenborn, W.D. 1996. Salt cedar impacts on salinity, water,fire frequency, and flooding. In: Proceedings of the Salt cedar Management Workshop. Holtville, CA: University of California Cooperative Extension, Imperial County: 9–12.